Back in the bad old days of VOR navigation you could find your position by tuning in two different VORs. Each radial from a VOR was a “line of position” from that VOR. Your position could be anywhere on that line. Where the two lines from two different VORs intersected was your location. For accuracy you had to plot the radials on a paper sectional, but it worked.
If you were lucky, you might have had a DME, meaning you only needed to tune one VOR for the line of position and use the DME for distance along that line. Plotting was easier that way. But either way, you needed the paper chart to see where you were and your accuracy was at best within a mile or so. These days, GPS-driven moving maps are ubiquitous—even available on some watches—and instantly show your position within a few meters. How can that be?
The Basics
To understand GPS positioning we must first realize that radio waves travel at the speed of light. The speed of light is constant at about 3.0 × 108 meters per second or 186,000 statute miles per second. Knowing how long it takes for a radio signal to get to us allows a simple calculation to tell us the distance from the source. If it takes 65 milliseconds for a signal to get to us from a satellite, that means we must be about 12,000 miles away from the satellite.
But that’s not enough. Just like with VORs we need at least a second source to help us figure our position. If all we know is how far we are from a single satellite, based on the time it took for the radio waves to reach us, then we could be that distance in any direction from the satellite. Given that the universe is three-dimensional we end up with a “sphere of position.” We could be anywhere on the surface of a sphere the given distance from the satellite.
Adding a second satellite gives us another “sphere of position.” We would be on the surface of that sphere of position as well as the surface of the sphere of position from the first satellite. Imagine that two satellites happen to be in positions such that their spheres of position just barely touch, with no overlap. If that were to happen, our position would be at that point where the spheres touched.
But generally we are not that lucky and the spheres of position do overlap. When two spheres overlap, the intersection of the surface of the spheres forms a circle. This set of points where the two spheres intersect is known as a “circle of position.” While this circle of position is a lot smaller than an individual sphere of position, it can still be a large area, meaning we don’t yet have a precise position.
If you’re following along, you’ve probably already guessed we simply need to add a third satellite. With the sphere of position from that satellite, we now have a good estimate of our position— if you intersect the sphere of position from the third satellite with the circle of position from the first two satellites, you end up with just two points. One of those two points will be out in space so that answer is thrown away. The result is our place on earth.
If we add a fourth, fifth or more satellites, we can determine our position with even more precision. That’s why it is generally said that we need a minimum of four satellites in view to get a good position fix.
GPS satellites are not in geosynchronous orbits—that is they appear to an earth-based observer to be moving across the sky. This means that if there aren’t enough satellites in orbit, there might be times when there are only one or two satellites overhead. A minimum of 24 satellites is needed to insure that there are always four or more satellites in view at any point on the surface of the earth.
Fewer satellites could be used if they were in a fixed orbit making them stationary over the United States—a geosynchronous orbit. There are three problems with this. First, geostationary orbits require the satellites to be much further away from the earth. This requires much higher power transmitters on the satellites, making them heavier, larger, and ultimately much more expensive. Then, the angles involved wouldn’t always produce a good solution to the position equations. Finally the GPS system was developed, funded, and maintained by the Department of Defense for the ultimate purpose of being able to operate anywhere in the world, not just in the United States.
Precise Timing
But all of this math is dependent on knowing exactly how long it took the radio signal to travel from the satellite to our receiver. To get that timing, we must start by knowing precisely when the satellite sent the signal, where the satellite was when it sent the signal, and when we received it. That’s where “ephemeris” data comes in.
Each satellite knows its own position. It also has an onboard atomic clock. Each satellite continuously transmits this information. Each GPS receiver—from the panel-mounted navigator in your airplane to the sports smartwatch on your wrist—collects this data from all the satellites in view. It then averages the clocks from all the satellites to get an extremely accurate time of day. Since the ephemeris data includes the position, orbital path of the satellite, and the time the signal was transmitted, your GPS receiver can use the data to calculate its sphere of position from the satellite.
The math required for all this gets quite complex and for maximum accuracy involves Einstein’s Theory of Relativity. Forty years ago, the calculations were complex enough that a handheld receiver would not have been able to keep up. But as the semiconductor industry advanced, so did compute power and these days we would be upset if even our smallest devices couldn’t determine our GPS position accurately.
However if you’re shooting an LPV approach to minimums, the accuracy of your phone might not be enough. The original GPS satellites had an accuracy of about 15 feet. More recent satellites can be as accurate as one foot. But these accuracies are typical, not worst case. Plus, of course there are still old satellites in use. For these reasons and more we need a way to augment the accuracy for approaches where we might still be in the clouds only 200 feet above the ground. Remember that any inaccuracy of your GPS receiver is added to the inaccuracy of your skills, altimeter, instrumentation, etc. It all adds up, so minimizing the inaccuracy of any one component, like the GPS signal is important. That’s where WAAS comes in.
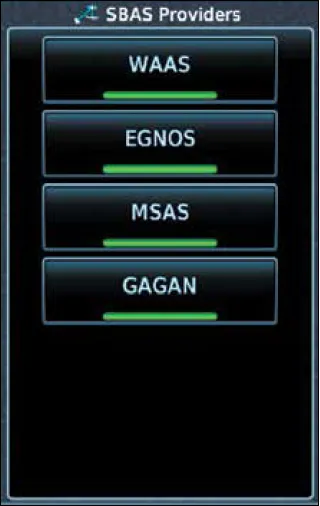
WAAS For More Accuracy
WAAS (Wide Area Augmentation System) is the FAA’s way to enhance GPS accuracy. WAAS operates by having ground stations (Wide Area Reference Stations—WRS) that receive the signals from the satellites. Each WRS is accurately surveyed so that it knows its own position before being placed into operation. It compares its known position to the position it computes from the satellites. It then computes the difference between the two positions.
The ground station then sends this information to a central collecting computer (Wide Area Master Station—WMS) that assembles the data from all the other WAAS ground stations. That information is then transmitted to satellites that are in a geostationary orbit over the United States. (Yes, WAAS is only available in the U.S.) Those WAAS satellites then send the error-correction information down to your receiver.
Your receiver applies this error-correction information to the calculations to give you a much more accurate position solution. The additional information increases the reliability of the solution as well.
Your receiver checks the availability and reliability of the signals that it is receiving. If it detects that the incoming signals are not good enough for a reliable calculation of your position it will flag a RAIM (Receiver Autonomous Integrity Monitoring) error. For a full discussion of RAIM, see the excellent article, “Revisiting RAIM” by Fred Simonds in the August 2021 issue of IFR.
Can You Use It?
Even if your receiver is able to determine your position, you need to determine if your receiver is legal to use for IFR flight. For VFR flight anything is legal to use for navigation under Part 91, whether you choose a paper chart, an iPad, or even a ham sandwich! But there are many rules about the legalities of using GPS for IFR flight under Part 91.
The first and most basic rule is that the receiver must be permanently installed in the aircraft. iPads and cellphones can be used for supplemental information or in the case of an emergency, but they cannot be used as a primary source of IFR navigation.
To be legal as a primary source of navigation under IFR, the GPS must certified as a TSO C129 (non-WAAS) or TSO C145/146 (WAAS) receiver. Additionally the database must be current. There are some manufacturer-specific exceptions (Check your flight-manual supplement.) for using an expired database for en-route and terminal operations but instrument approaches are not allowed with an expired database. There used to be a requirement that database updates be logged but this requirement was eliminated years ago.
Additionally you must have the Cockpit Reference Guide (not the User Manual) in the cockpit and reachable by the pilot for the unit to be legal to use. Lots of aircraft don’t have the Guide in the plane, so that’s something to check. Finally when the unit is installed in the plane, it must be functionally tested in flight and the results of the test must be sent to the FAA and entered into the aircraft maintenance logs before it is legal to use under IFR. I have flown a few planes where this entry was missing from the logs. If missing, that GPS is not legal for IFR.
Once the unit is properly installed in the plane and a current database is loaded to the unit, it’s important to know when and where you can substitute the GPS for terrestrial navigation sources. AIM 1-2-3 is the place where we can find out what the FAA allows in terms of GPS substitution. After all is said and done, the simplest way to remember what is allowed and what is not allowed is the following: GPS can always substitute for any land-based navaid with two exceptions. 1) On the final approach segment (from the FAF to the MAP and 2) anywhere on a localizer- based approach. Other than these two exceptions you can substitute your GPS for a VOR, DME, or NDB anywhere or anytime you would like.
Knowing how a GPS operates and what makes it legal might not make you a better pilot or improve your proficiency. But just like knowing how the engine works in your aircraft makes you better prepared for emergencies, knowing how your GPS works can be very helpful in unusual situations or, worse, with failures. Be sure to learn as much as possible about your GPS and you will be a better pilot, even more comfortable with GPS navigation.
This article originally appeared in the September 2021 issue of IFR magazine.
For more great content like this, subscribe to IFR!
The comment regarding the use of GPS as a substitute for the final approach segment of a VOR approach is incorrect. Since May 2016, suitable GPS units ARE allowed to be used for the final approach segment, provided the underlying VOR signal can be monitored. It cannot be used if the VOR is inoperative. Reference: AIM 1-2-3, para c, note 5: “ Use of a suitable RNAV system as a means to navigate on the final approach segment of an instrument approach procedure based on a VOR, TACAN or NDB signal, is allowable. The underlying NAVAID must be operational and the NAVAID monitored for final segment course alignment.”
“ Each satellite knows its own position.” How does the satellite know it’s position?
I didn’t see an explanation in the article.
OK, I found the answer.
“GPS satellites don’t have a computer that calculates its position by itself. GPS satellites transmit their clock information. GPS ground network use the clock received by multiple stations over a long period to calculate the GPS satellite’s orbit very precisely and use it to populate the GPS almanac and ephemeris.
https://www.quora.com”