Maneuvering speed is something fledgling airplane pilots learn about early in their training. It’s one of the more important details to know about any airplane because it has implications for structural integrity. It’s also relevant to operating in turbulence and performing maneuvers, although its value isn’t on an airspeed indicator, mainly because it varies with weight.
Maneuvering speed was taught to many pilots as the speed above which rapid, full control movements must not be made. The implication—and one of the lessons many pilots drew—is that rapid, full control movements could be made below that weight-adjusted speed. Then came American Airlines Flight 587, an Airbus A300 that crashed shortly after departing New York’s John F. Kennedy International Airport on November 12, 2001, killing all 260 aboard and five on the ground.
Investigation revealed the Airbus encountered the wake turbulence of a Japan Airlines Boeing 747 that took off less than two minutes earlier from the same runway. To counter the wake turbulence, the Airbus’ first officer and pilot flying made multiple, rapid left and right rudder inputs. This snapped off the jet’s vertical stabilizer and the airplane entered a flat spin from which it did not recover.
The Airbus was below its maneuvering speed when its vertical stabilizer failed, which mystified many pilots, given their understanding of the value’s relationship to structural integrity. What few pilots had considered, however, was that the rapid pulsing of the rudder and the resulting sideslips placed loads on the components well above what their engineering allowed.
The lesson many pilots learned was that flying below maneuvering speed only ensures airframe integrity when a single, full control deflection is made. When rapidly making opposing inputs, all bets are off. Apparently, even with that nuanced understanding of maneuvering speed, some pilots may think it doesn’t apply to them.
Background
On June 1, 2017, at about 1155 Pacific time, a Cessna 180 experienced an in-flight breakup and was destroyed when it impacted terrain near Ventura, Calif. The 52-year-old solo private pilot was fatally injured. Visual conditions existed along the airplane’s route from Santa Paula, Calif.
A witness heard a loud sound, looked up and saw the airplane spinning towards the ground. The engine and both wings had separated from the airplane. Its tail was still attached.
The main wreckage was on a mountaintop at about 1015 feet MSL and consisted of the fuselage starting from the nose and ending just short of the vertical stabilizer. Most of the airplane debris was within about ½ mile of the main wreckage. A section of the right wing was found about 1.5 miles from the main wreckage. All major components of the airplane were in the debris field.
Investigation
The inboard left wing was found about 295 feet southeast from the fuselage. The empennage, including the horizontal stabilizers, vertical stabilizer, left elevator, rudder and tailwheel, was about 455 feet from the fuselage; the right wing was located about 420 feet from it. The engine and propeller were about 510 feet from the fuselage. The engine revealed no evidence of any pre-impact malfunction precluding normal operation.
Both wings failed in a manner consistent with a negative overload failure. No corrosion or pre-existing cracks were observed at the spar fractures. The empennage displayed multiple deformations and fractures. Damage to the right stabilizer and elevator were consistent with a significant download that exceeded the capabilities of the structures. The left horizontal stabilizer and elevator likely were damaged by a partial downward failure. All fractured flight control cables had a splayed, broomstrawed appearance, consistent with tension overload, but were intact before the breakup.
The airplane’s weight and balance records were not located. Based on the pilot’s weight, presuming a 55-gallon fuel load and factory information, the airplane would have been several hundred pounds below its maximum gross weight of 2550 pounds and within its CG limits at the time of the accident. The airplane’s published maneuvering speed (VA) was 122 mph (106 knots).
An airplane performance study used radar data to show the airplane was flying steadily in the minutes before the breakup. The airplane gained speed toward the end of the data, with groundspeed increasing from 82 to 127 knots over 67 seconds. The calculated maximum airspeeds were higher than the maneuvering speed.
Weather observed at a sea-level station around the time of the accident about 10 miles southeast of the wreckage included wind from 240 degrees at seven knots, visibility of 10 statute miles and scattered clouds at 2300 feet. No significant weather or turbulence was reported or forecast in the area at the time of the accident, although modeling estimated a high probability of moderate or greater turbulence between 2300 feet MSL and 3300 feet MSL.
A year earlier, the pilot reported accumulating some 1100 hours of flight time on his medical certificate application, with 50 hours in the preceding six months.
Probable Cause
The NTSB determined the probable cause(s) of this accident included: “The pilot’s abrupt and substantial pullup maneuver while flying the airplane above the maneuvering speed, which resulted in an in-flight breakup. The reason for the pilot’s maneuver could not be determined based on the available evidence.”
Without knowing the cruise altitude, it’s hard to dismiss the possibility of the airplane encountering turbulence. But, according to the NTSB, the radar data indicates it’s likely “the pilot performed an abrupt and substantial pull up maneuver, while flying the airplane above maneuvering speed…. It could not be determined why the pilot would suddenly pull up….”
It’s clear from the radar data that it was flying above its maneuvering speed at the time. Whether an abrupt control movement, or movements, or unreported turbulence was the culprit is something we’ll never know. Regardless, it’s clear the airplane came apart when it probably shouldn’t have. And this gives us all another reason, as if we needed it, to heed limitations like maneuvering speeds.
Aircraft Profile: Cessna 180
OEM Engine: Continental O-470-A
Empty Weight: 1540 lbs.
Maximum Gross Takeoff Weight: 2550 lbs.
Typical Cruise Speed: 137 KTAS
Standard Fuel Capacity: 55 gal.
Service Ceiling: 21,200 feet
Range: 513 NM
VS0: 51 KIAS
The Envelope, Please
Pilots often talk about “exploring” or staying inside “the envelope.” They’re talking about the Vg diagram below, which exists for any airplane. The diagram explains the relationship between velocity and G loading, or load factor.
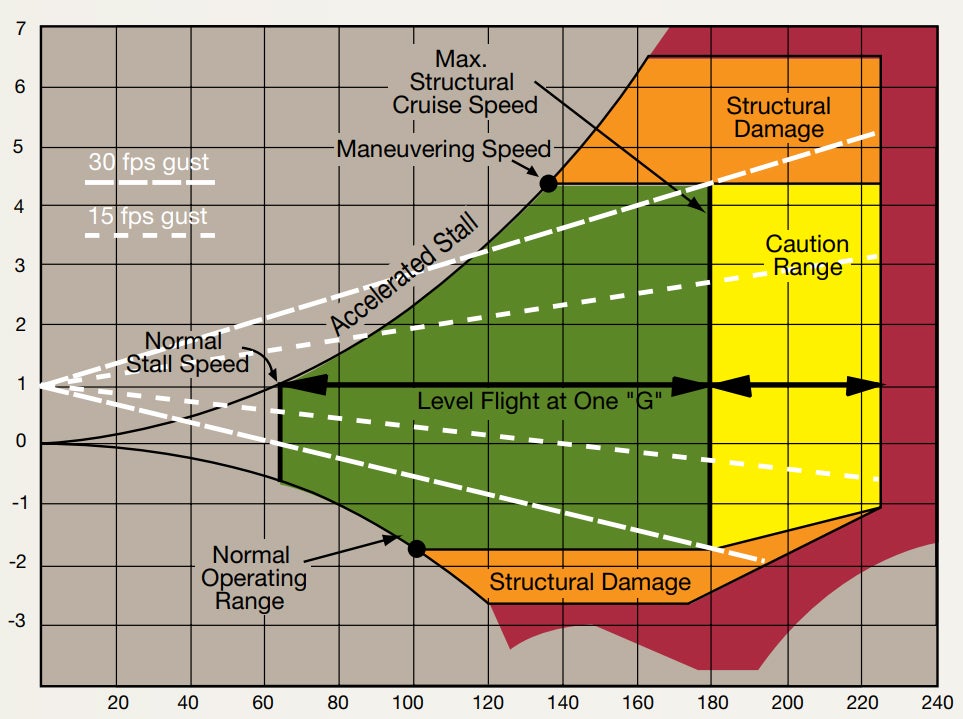
When considering maneuvering speed, it’s important to remember that an aircraft operating below it cannot be damaged by a positive flight load factor in excess of its designed strength. It will stall first. Similarly, combining a gust with abrupt maneuvering also won’t result in damage below that speed. But when we exceed maneuvering speed, which usually isn’t hard to do, an otherwise benign control input coinciding with a gust at the wrong time can combine for an undesired outcome.
Jeb Burnside is the editor-in-chief of Aviation Safety magazine. He’s an airline transport pilot who owns a Beechcraft Debonair, plus the expensive half of an Aeronca 7CCM Champ.
This article originally appeared in the June 2020 issue of Aviation Safety magazine.
For more great content like this, subscribe to Aviation Safety!
One should be aware that the v-n-diagram assumes stationary conditions, i.e. the changes in angle of attack are slow. This will ensure that the plane will stall before exceeding its structural strength below maneuvering speed. However, for high angle of attack rates (i.e. abrupt control deflection), the airflow may still be attached for a short time although the stationary angle of attack has already been exceeded. This is known as dynamic stall, a problem which was first encountered in helicopter blades and is a current research topic for wind turbine airfoils.
During the short time of lift overshoot, the lift force continues to increase linearly as if no stall was present and then it suddenly drops far below the stationary stalled value. The strength of the lift overshoot and successive drop increases with angle of attack rate. The effect is usually negligible for general aviation aircraft with low maneuverability (i.e. non aerobatic), but it may make a difference when an abrupt control deflection and/or angle of attack change due to a gust occurs when the airplane is already flown close to its limits.
Contrary to the brief summary above, it’s worth reading the Wikipedia article on the Flight 587 accident
for the nuances into how the AA sim training exercise was at fault, and how pilots had no idea that just two consecutive rudder inputs could snap off the tail:
https://en.wikipedia.org/wiki/American_Airlines_Flight_587
Adding to that I would suggest that we also consider the direction of a gust load when it affects the aircraft. I used to fly a PA46 and attended a number of seminars run by John Mariani who was closely involved with the original design and certification of the PA46. This particular type had a higher than expected in flight break up rate after it was certified leading to a special certification review by the FAA – which essentially exonerated the aircraft but pointed to pilot awareness and training as a contributory factor.
According to the TCDS (I looked at the EASA one) the Va is 135kts at 4100lb coming down to 103kts at 2350lb – a rather clear demonstration of the effect of weight on Va.
PA46’s tend to fly high where there is a higher risk of running into significantly turbulent vertically developed weather. Vertical gusts of 3000 fpm are quite common, 5000 fpm not out of the ball park. 5000fpm is 50kts (close enough).
Consider a pilot who has reduced speed to maybe 120 knots – below the Va for that weight. The aircraft is then hit by a gust from ahead and below (gusts are not just vertical or horizontal – they can come from any direction). The worst case scenario is a heavy gust hitting the aircraft angled upwards at 45 degrees toward the nose. We can resolve the 50kt gust @ 45 deg into a vertical and horizontal component. Both the sin and cos of 45 deg is approx 0.71. So in the instant the gust hits the instantaneous airspeed increases to 120 + 50 x 0.71 = 156kts. At the same time the aircraft is hit from below by a 50 x 0.71 = 36kt gust.
A millisecond before this happened the pilot thought they were well within Va. A millisecond later, through no fault of their own, they weren’t.
That’s why JM taught, in his classes, that if we hit severely turbulent weather – SLOW DOWN. Not to Va, but well below. He recommended about 100kts in the 46 – and put the gear down. The gear is down to act as an airbrake in the case of momentary loss of control which could lead to an overspeed. Not flap by the way – because that decreases the stall speed and this is exactly what you DON’T want. If the aircraft does hit a heavy gust and gets a short duration stall then that’s something we can deal with with a standard stall recovery technique. Just reduce the AoA as required to install the wing.
But that control input is only possible if the wings and empennage are still attached to the airplane. Going the other way risks structural failure of something needed to keep the airplane flying. And that is always going to end up with someone having a very bad day.
As the article says this is not isolated to our little aircraft – there are documented examples of pilots pulling the wings off PC12’s, TBM’s etc.
I experienced a particularly sobering incident many years ago while descending in a Cessna 404 from over mountains into Boulder, Colorado with a strong tailwind at altitude. This was before the days of GPS, so I can only presume that I had an exceptionally high ground speed due to the strong tailwind. I observed clouds ahead that indicated possible rotor activity, so I slowed well below Va in anticipation of turbulence. I was shocked by the violent turbulence and wild airspeed fluctuations that I experienced.
I remember reading of the in-flight breakup of a Malibu during descent at night, after passing a mountain ridge with a strong tailwind. I wonder if anyone has considered or studied the possible hazards associated with descending on the lee side of mountainous terrain with a strong tailwind, high groundspeed, and then suddenly encountering a stationary rotor, with air flow suddenly shifting from a 50 knot tailwind to a 50 knot headwind on the bottom of the rotor. It seems to see that the sudden increase in airspeed and possible violent up and down gusts could easily destroy an airplane. I recall a turbine Aero Commander coming apart under similar circumstances near Denver in the 1980’s.
Question about the wording of this sentence:
>Both wings failed in a manner consistent with a negative overload failure
Does “negative” refer to a negative g loading? The rest of the report seems to indicate positive g due to the pilot’s rapid pullup. Clarify please? Thanks.
Chris – I had the same question. Some clarification by the author will be helpful. It takes less speed, less control force to exceed structural limits in negative Gs.
I’m surmising that rapid pull-up (or gust) put too much down-force on the horizontal stabilizer, causing it to fail downwards. As the report stated:
“Damage to the right stabilizer and elevator were consistent with a significant download that exceeded the capabilities of the structures. The left horizontal stabilizer and elevator likely were damaged by a partial downward failure.”
Once the horizontal stabilizer departed the aircraft, the normal down-force that is present in cruise flight went away. This caused the aircraft to rapidly pitch forward, presenting the top surface of the wings broadside to the airflow from forward motion. The wings then failed ‘negative’ or downwards relative to the fuselage. The aircraft at that moment was pointed nose down but still moving horizontally, causing the wings to fail ‘backwards’ relative to its forward motion.
From articles that I have read over the years, it seems that during a high-g, high speed, in-flight breakup, the horizontal stabilizer fails downward first, causing an instant pitch forward that fails the wings in a negative direction.
If the aircraft could not withstand a series of successive maneuvers,
then it was poorly designed, inadequately tested, and hazardous to
fly, regardless of anything else. The manufacturer is either grossly
incompetent, criminally negligent, or both. That conclusion is what
follows from the detailed reports and expert analyses given above.
All that remains is for us to draw it, make it explicit, then act on it.
A good article, but like so many other articles on the same topic, it does not mention what happens to Va if the airplane is modified by STC or other means to reduce the stall speed of the aircraft. And in articles reviewing such mods, like adding VGs or other stall-reducing tricks, I don’t see any discussion of what happens to Va in those either. I would think in any article addressing the importance of Va relevant to inflight breakups, or in any article addressing STOL mods, there should be some comment regarding how the Va will (or in the case of a mod, HAS ALREADY) change the Va due to the reduced stall speed. Of course, a lowered stall speed after an STOL mod means that the structure may fail even if the pilot flies at a speed below Va, even if he takes into account how much his gross weight might affect the POH Va speed.
The failures are consistent with empennage failure followed by pitch down exceeding negative load limits for the wings.
Both wings and engine departed the airplane.. This extreme inflight damage had to have had a “yanking reaction” on the controls.. The point of “airspeed control” has been driven home to the “umpth degree”, yet smooth control inputs is what stands out from my perspective..