If you studied aviation accident reports as much as we do, one of the many phrases you’d often see is “failure to control airspeed.” It crops up in runway overruns a lot, but primarily appears when discussing in-flight loss of control accidents, of which there are enough the probable cause has earned its own acronym: LOC-I. The classic LOC-I accident might involve a stall/spin event at the low end of the airspeed indicator or airframe failure at the other. But the bottom line is someone wasn’t paying attention to a major thing that’s critical to controlling the airplane.
As the saying might go, “Everyone talks about airspeed control but no one does anything about it.” Let’s try to chip away at that problem for a moment, by highlighting the four basic ways we control airspeed—power, pitch, drag and trim. Also important is how they interact and how managing all of them is the only way to ensure we’re using all of the controls, including the ailerons and rudder, to achieve coordinated flight.
Power
Power’s relationship to airspeed control usually is well-understood: Increase power to go fast, reduce it to go slow. That simple cause-and-effect relationship falls down, however, when we’re behind the so-called “power curve,” which is explored more fully in the sidebar “Where Are You On The Power Curve?” below, and which also highlights the need to coordinate other control inputs to manage airspeed.
As we change the power setting, a typical airplane will want to change its pitch attitude. The rule of thumb is that an airplane with its thrust line roughly in alignment with the center of gravity (CG)will suffer little or no tendency to change pitch as the power setting is changed. As we move the thrust line vertically away from the CG, the airplane’s tendency to change pitch is increased. When the thrust line is above the CG, the airplane will tend to pitch down when power is increased, and vice versa. Also involved in this tendency is the extent to which, if any, a power change alters the flow of air over the typical airplane’s horizontal stabilizer. When we change power but want to remain at the same altitude, we must change the airplane’s pitch attitude.
Pitch
Like power, the airplane’s pitch attitude has a huge impact on its airspeed. As an example, for a given power setting, there are pitch attitudes that produces a descent and others resulting in a climb. That’s one reason pilots maintain that power has little impact on airspeed but pitch has everything to do with it. The truth—and one of the major points underlying this article—is that they are related and depend on each other to be managed by the pilot to achieve the desired performance.
On one of your first flying lessons, the instructor probably demonstrated the relationship of pitch to airspeed. At a constant power setting, she directed you to pull back on the pitch control. In response, the airplane entered a climb and airspeed decreased. When the change in altitude and speed was apparent, she then asked you to push forward on the pitch control, beyond level flight. The result was a descent, while airspeed increased. That demonstration should have made very clear that airspeed is dependent on power and pitch, and that neither control has absolute authority over airspeed.
Drag
Often overlooked in discussions of airspeed control is the impact drag can have. This is relatively easy to demonstrate in almost any personal airplane. To do so, start in level flight at an airspeed below the maximum flap extension speed—below the top of the airspeed indicator’s white arc—and add a notch of flaps. One thing is certain to happen: the airplane will slow down, maybe not immediately, but soon. In the bargain, its pitch attitude may change. To slow further at the same power setting, add another notch of flaps. By this point, you’ll likely have to change pitch to maintain altitude, but airspeed will have been reduced even further from its no-flaps value.
The same effect drag has may be demonstrated by lowering the landing gear in a retractable or, for a demonstration with much less magnitude, opening the cowl flaps on an airplane so equipped.
Trim
Often omitted from airspeed-control discussions is the use of pitch trim. That’s because, like drag, trim is considered a secondary control. We typically use it to reduce pilot workload, especially in cruise flight with constant power and pitch. In a way, it works to verify the relationship between power and pitch.
The demonstration is this: From trimmed cruise flight, reduce power. The airplane will begin to descend at the same airspeed to which it was trimmed. Alternately, establish a trimmed, full-power climb, then reduce power. The airplane will slow to its trimmed airspeed and the climb rate, if any remains, will be minimal. The reverse is true if adding power in a trimmed descent
The bottom line in all this is that controlling airspeed is not a matter of using one control, whether primary or secondary. Instead, it’s an “all together now” thing, and coordinated flight requires us to use all the available controls.
Which Airspeed
Whenever we talk about controlling airspeed, we’re talking about indicated airspeed. But there are two other kinds of airspeed we typically need to concern ourselves with.
Indicated airspeed
IAS is the direct instrument reading obtained from the airspeed indicator, uncorrected for variations in atmospheric density, installation error or instrument error.
Calibrated Airspeed
CAS is IAS corrected for installation and instrument error. At certain airspeeds and with certain flap settings, installation and instrument errors may total several knots, and generally is greatest at low airspeeds.
True Airspeed
TAS is CAS corrected for altitude and non-standard temperature. Because air density decreases with greater altitude, the ASI will indicate lower than “true” because there is less difference between pitot impact pressure and static pressure.
Where Are You On The Power Curve?
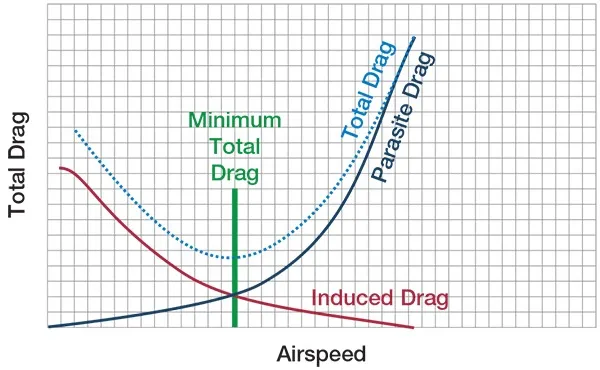
When pilots talk about the power curve, they’re talking about a graph like the one above. The graph plots airspeed on its X axis and total drag on the Y. Total drag, of course, is the sum of induced and parasite drag. Parasite drag, meanwhile, increases with airspeed while induced drag’s increase corresponds to the amount of lift generated. For every airplane at a given weight and configuration, there is a certain airspeed that can be called the “minimum drag speed” at which total drag is the least it can be in flight. This point is marked on the graph above by the green vertical line.
The minimum-drag speed is important in another way. When we accelerate above that speed, drag increases and the airplane is not flying as efficiently as it could, at least as far as drag is concerned. Meanwhile, when we slow the airplane below that speed, induced drag increases, requiring more power to maintain altitude even as airspeed drops. The front of the power curve is the area to the right of the green line; the area to the left of the green line is behind the curve.
This article originally appeared in the December 2020 issue of Aviation Safety magazine.
For more great content like this, subscribe to Aviation Safety!
With more and more PFDs and MFDs on the panel who has time to monitor airspeed with all that cool data to be scrolled through. Pilots now have too little focus and far too much information.
Seagull, don’t forget those GoPro cameras you have to manage too. Tik Tok pilots.
I’m sure they all have 337’s in the A/C logbook.
A critical point that is not mentioned, is needing to keep sufficient airspeed margin above the stall speed, for the aircraft situation.
Departure stalls due to excessive pitch and decreasing airspeed leading to the critical AOA.
Accelerated stalls during a turn in the pattern can also cause exceeding the critical AOA.
Most POH’s have data on both situations for safe airspeed margins vs weight and bank angle.
This is yet another article on pitch and power that’s in fantasy land.
“establish a trimmed, full-power climb, then reduce power. The airplane will slow to its trimmed airspeed”. This is miswritten. If the airplane is established in a trimmed out climb, and power is reduced, the angle of attack will decrease due to less downward pressure on the tail. The tail moves up because it is keeping equal pressure above and below it. This will keep approximately the same airspeed. The airplane doesn’t slow to its trimmed airspeed – it keeps its trimmed airspeed.
“The reverse is true if adding power in a trimmed descent”. This depends. If you add power on a go-around, you know this is totally false – it will not keep its trimmed airspeed. Most of the time, the airplane will pitch up far too much, lose airspeed and stall.
“when we slow the airplane below that speed (minimum-drag speed), induced drag increases, requiring more power to maintain altitude even as airspeed drops.” This is the big fantasy – that power controls altitude. In a V22 Osprey – yes. The huge engines and rotors tilt up and create a vertical force that can exceed the weight. It converts into a helicopter. With an airplane, the thrust line is fixed in a horizontal position. The maximum force created by the propeller is a small percentage of its weight (about 500lbs in a Skyhawk which weighs over 2000lbs). Every time you perform a full power stall, you are proving that the power can’t control the altitude. Many pilots are, however, confused about this on approaches. They get established on approach and trim out. Then they keep the airplane (single-engine) on the glideslope by increasing or decreasing the power. Why does that happen? It changes the propeller slipstream and downward force on the tail, changes the angle of attack, and changes the lift vector, the actual force that opposes weight. However using a cushion of air on the tail is a poor way to control angle of attack – pressure on the control wheel is far more effective and safer and works in all conditions. When it comes time to flare, normally power is reduced to idle and the flare is done solely with the elevator. That also proves that power doesn’t control altitude – if it did, the airplane would fall onto the runway when power is reduced to idle.
With that said, coordination is key. If more lift (back pressure) is needed to correct for being low, or to flare, speed awareness is critical. Power will have to be simultaneously increased if you don’t want to also lose airspeed. Most of the time, the pitch and power adjustments are quite small but adjust as needed. And it’s critical to understand basic limitations – it isn’t possible to increase pitch in an unlimited manner which will cause a loss of speed even with full power or cause a stall. And don’t forget what full power at that point can do if you don’t apply forward pressure on the control wheel.
JimH in CA got his wires crossed with the statement, “Departure stalls due to excessive pitch and decreasing airspeed leading to the critical AOA”. It is exceeding the critical AOA in any attitude and/or at any airspeed which causes the stall.
The main article stated that there are three airspeeds, Indicated, Calibrated, and True. Actually, there is a fourth – Equivalent Airspeed which accounts for compressibility. Not many GA aircraft get into high mach, subsonic aircraft, but it is there. Here’s a good description: https://en.wikipedia.org/wiki/Equivalent_airspeed
You’re right Dcmarotta. But if the Aviation Safety Magazine has, as primordial readers, pilots of GA, may be the explanation and development of EA (equivalent airspeed) and respective implications, weren’t necessary for those readers. Perhaps just a single note to inform about that airspeed and that the article, for the proposed matter it was written, didn’t develop.
This article is an excellent primer except that angle of attack was not mentioned once. Yet AOA encapsulates everything mentioned in the article and more. Why GA pays such little or no attention to angle of attack is puzzling.
Where am I on the power curve? A simple glance at my homemade AOA indicator consisting of a vane with a pointer on a half round scale fastened to the underside of my wing forward of the leading edge tells me everything I need to know about where I am on the power curve. The scale has two markers: stall and Vx. The airplane stalls when the pointer points to the stall marker. When the pointer is pointing at the Vx marker, the airplane is climbing at best angle of climb when at full power. With partial or no power applied while pointing at the Vx marker the airplane is either slow flying or descending at an optimal angle of attack for a short field landing. With the pointer pointing anywhere above the Vx marker, I’m safely ahead of the power curve.
Yes, failure to control airspeed causes accidents, but it’s really failure to control AOA which causes failure to control airspeed related accidents. If AOA was taught on every primary training flight as a basic I’m convinced there would be fewer failure to control airspeed accidents.
Oh, and which airspeed? If you fly the AOA, which airspeed whether calibrated, indicated or true becomes irrelevant knowledge in the moment.